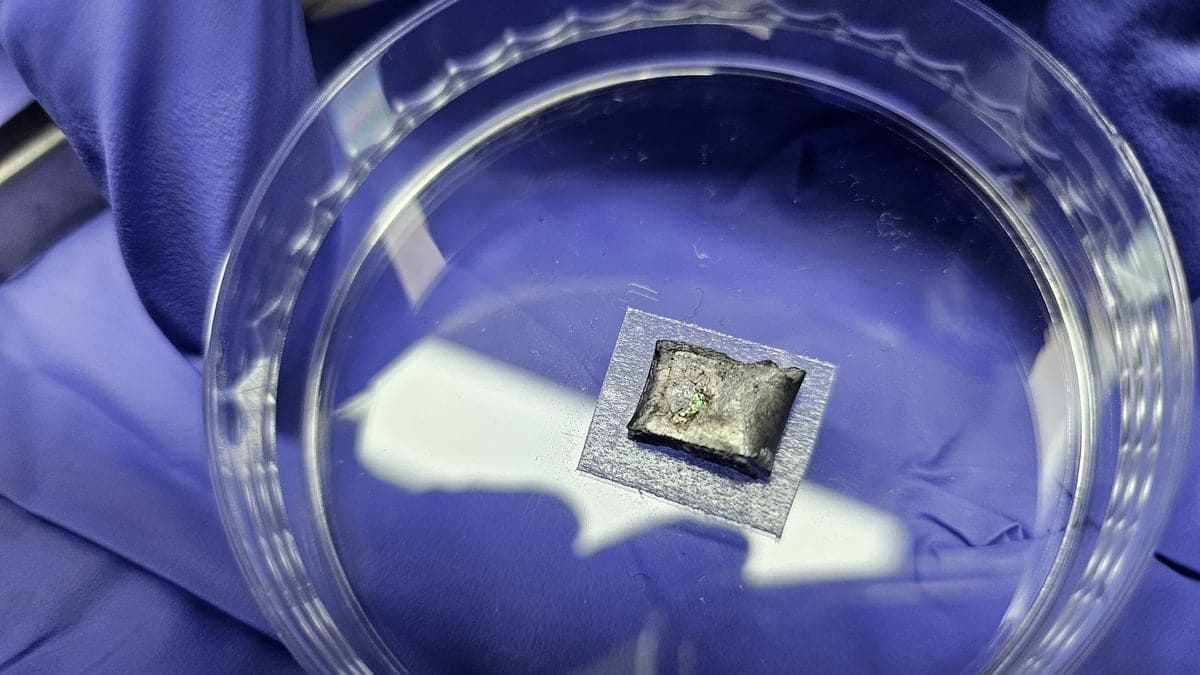

Within a laboratory in South Korea, a graduate student’s attention was drawn to a mesmerizing rainbow shimmer. This stunning effect is the result of an incredible scientific breakthrough: diamonds formed not under extreme geological pressure found deep in the Earth, but through a unique procedure using a liquid metal at atmospheric pressure. These innovative synthetic diamonds could revolutionize the production of one of the most prized and hardest materials known to mankind.
Redefining Diamond Creation
Traditionally, natural diamonds develop in the upper mantle of the Earth, where temperatures can exceed 900–1400°C and pressures soar to 5–6 gigapascals—thousands of times the atmospheric pressure at sea level. Since the mid-20th century, researchers have been able to recreate these extreme conditions using high-pressure, high-temperature (HPHT) techniques to generate synthetic diamonds.
However, a research team led by Rodney Ruoff at the Institute for Basic Science in Ulsan, South Korea, has overturned this conventional wisdom by successfully creating diamonds at just 1 atmosphere of pressure (the same pressure as sea level) and at a temperature of 1,025°C, utilizing a liquid metal alloy.
This groundbreaking journey began with a 2017 study that demonstrated how liquid gallium could promote the formation of graphene from methane at lower temperatures within a specialized vacuum setup. Intrigued by this discovery, Ruoff’s team hypothesized whether gallium could also stimulate diamond growth. Initial experiments involved seeding diamond crystals on silicon-doped gallium, although the results were inconsistent.

During one of their experiments, graduate student Yan Gong observed the formation of microscopic pyramidal shapes on the edges of a diamond crystal. “This made us realize that silicon played a crucial role,” recalls Ruoff. However, attempts to increase silicon content resulted in silicon carbide rather than diamond formation.
Persevering with their investigations, the team began experimenting with a novel liquid metal alloy combining gallium, iron, nickel, and silicon. After countless adjustments to the parameters, they made a breakthrough—Gong vividly remembers the day: “I spotted a ‘rainbow pattern’ spreading a few millimeters across the bottom surface of the solidified liquid metal. We discovered that these rainbow colors indicated the presence of diamonds!”
Understanding the Science behind Diamond Formation

The team’s innovative liquid metal technique involves subjecting the alloy to a combination of methane and hydrogen at a temperature of 1,025°C. Carbon from the methane penetrates the liquid metal, accumulating in a thin amorphous subsurface layer. This carbon-rich layer, combined with silicon, becomes the foundation for diamond nucleation.
“Approximately 27% of the atoms at the surface of this amorphous layer were carbon atoms,” notes co-author Myeonggi Choe. High-resolution imaging confirmed that diamonds form and grow within this layer, eventually coalescing to create a continuous diamond film.
Initially, small isolated diamond crystals began to appear just 15 minutes into the growth process. Over time, these crystals increased in size and merged into an uninterrupted film. By the 150-minute mark, the researchers had succeeded in creating a nearly complete diamond film, with only a few minor gaps remaining.
Theoretical analyses indicate that silicon stabilizes small carbon clusters, which serve as “pre-nuclei” for diamond development. Without the presence of silicon, diamond formation did not occur, highlighting its role as a critical catalyst in the crystal growth process.
Significance and Future Considerations
The ramifications of this discovery could be significant. For one, it may lower the barriers to diamond synthesis, making it more affordable and accessible. Conventional HPHT methods demand costly equipment and high energy consumption, while this new technique allows for diamond production at room pressure and relatively lower temperatures, potentially reducing costs and energy needs. Given diamonds’ outstanding thermal conductivity, hardness, and electronic capabilities, they are ideal for applications in advanced electronics, quantum computing, and medical devices.
Nevertheless, numerous questions remain unanswered. What makes this particular metal combination so effective? How stable are these synthetic diamonds, and can the process be refined to produce larger, purer specimens? Ruoff expresses optimism, stating, “There are a multitude of fascinating paths to investigate.”
The results of their groundbreaking research have been published in the journal Nature.
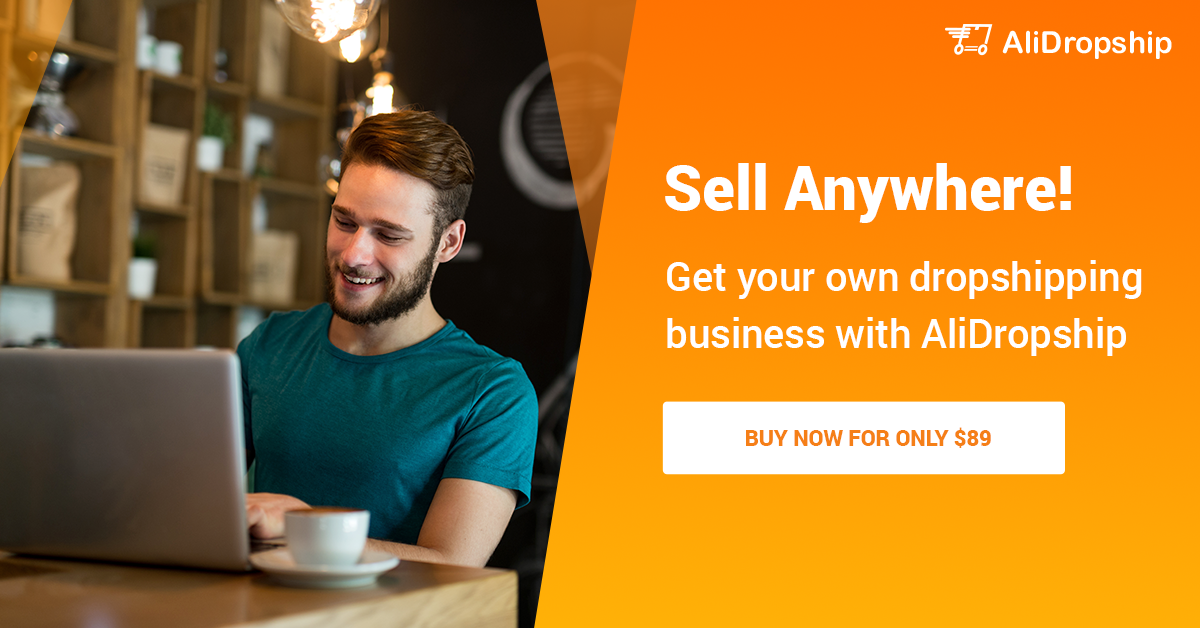