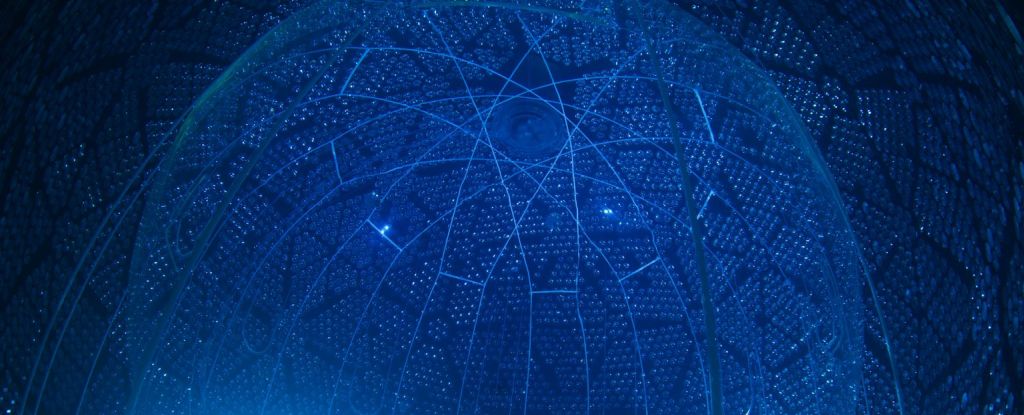
Deep beneath kilometers of rock in Ontario, Canada, a hidden reservoir of the purest water reacted as an elusive particle darted through its structure. This remarkable incident marked the first instance of utilizing water to detect an antineutrino, a particle that originated from a nuclear reactor located over 240 kilometers (150 miles) away.
This groundbreaking discovery, shared in a study published in 2023, lays the foundation for future neutrino research and innovative monitoring technologies that utilize affordable and safe materials.
Neutrinos are among the most plentiful particles in the Universe, exhibiting unique properties that offer insights into the cosmos. However, due to their nearly massless nature, lack of charge, and minimal interactions with other particles, they predominantly pass through matter, which is why they’re often referred to as ghost particles.
Antineutrinos serve as the antiparticle counterpart to neutrinos. Typically, antiparticles display opposite charges compared to their particle counterparts; for example, the positively charged positron is the antiparticle of the negatively charged electron. As neutrinos lack charge, scientists differentiate between them and their antineutrino counterparts by observing their interactions: an electron neutrino manifests alongside a positron, while an electron antineutrino appears with an electron.
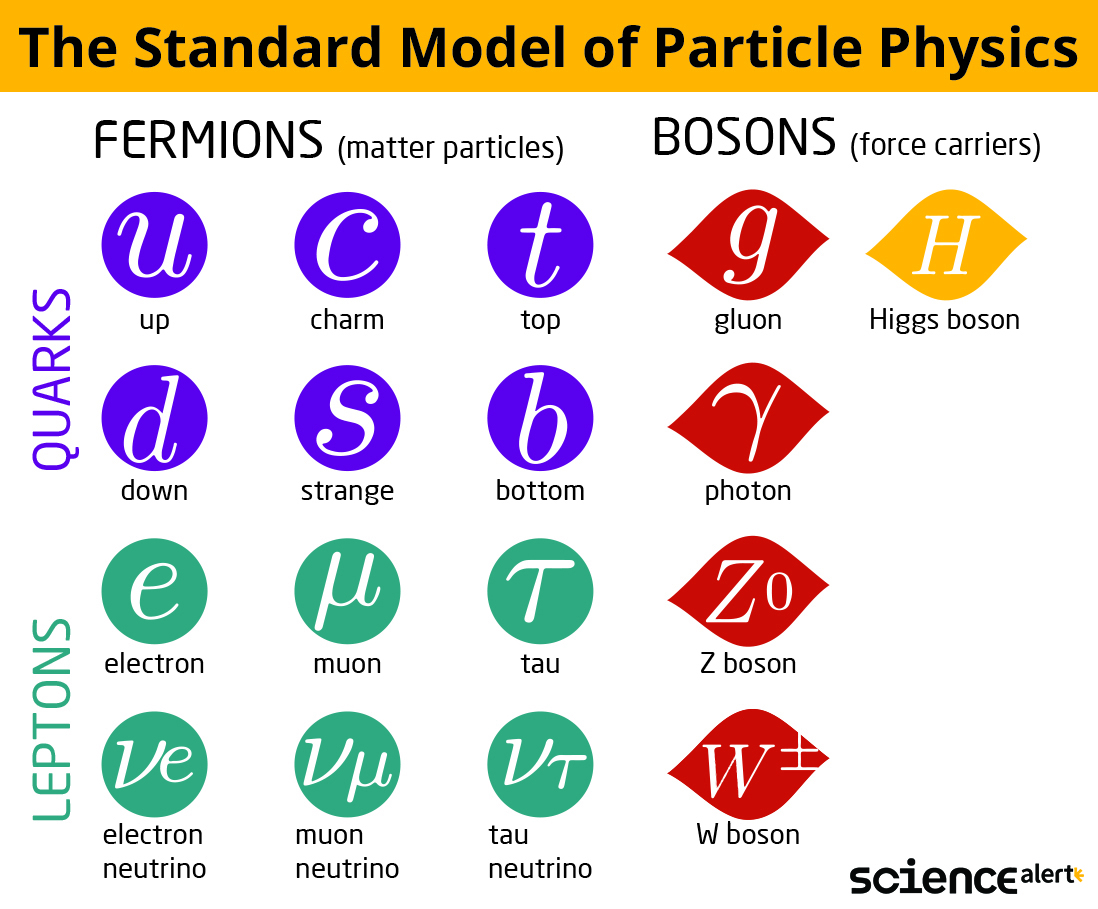
During the process of nuclear beta decay, electron antineutrinos are released. This decay occurs when a neutron transforms into a proton, an electron, and an antineutrino. An electron antineutrino can subsequently engage with a proton, resulting in a positron and a neutron, a phenomenon called inverse beta decay.
To detect this type of radioactive decay, enormous tanks filled with liquid and equipped with photomultiplier tubes are employed. These systems are expertly designed to capture the subtle light emitted by Cherenkov radiation, which occurs when charged particles travel faster than light in the liquid, similar to the sonic boom created when breaking the sound barrier. Consequently, they are highly sensitive to even the faintest light signals.
While nuclear reactors produce vast amounts of antineutrinos, their low energy levels make detection quite challenging.
Enter SNO+, the world’s deepest underground research facility, situated over 2 kilometers (1.24 miles) below the surface. This rock cover effectively shields the laboratory from cosmic ray interference, allowing scientists to obtain exceptionally clear signals.
Currently, the lab’s 780-ton spherical tank is filled with linear alkylbenzene, a liquid scintillator that enhances light detection. During the calibration period in 2018, this tank was temporarily filled with ultrapure water.
After analyzing nearly 190 days of data collected during that calibration phase in 2018, the SNO+ collaboration found evidence of inverse beta decay. In this reaction, the neutron generated captures onto a hydrogen nucleus in the water, producing a soft flash of light at a precise energy level of 2.2 megaelectronvolts.
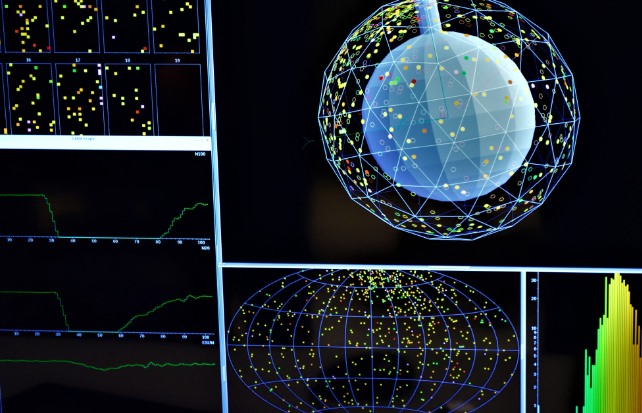
Water Cherenkov detectors typically struggle to identify signals below 3 megaelectronvolts; however, the water-filled SNO+ managed to detect signals down to 1.4 megaelectronvolts. This led to an efficiency rate of about 50 percent for detecting signals at 2.2 megaelectronvolts, prompting the team to pursue signs of inverse beta decay.
Upon analyzing a candidate signal, researchers determined it was most likely generated by an antineutrino, achieving a confidence level of 3 sigma, indicating a 99.7 percent probability.
This outcome suggests that water detectors could be employed to monitor the energy output of nuclear reactors.
In addition, the SNO+ facility is actively aiding in the enhanced understanding of neutrinos and antineutrinos. Due to the challenges in directly measuring neutrinos, their properties remain largely unknown, with one of the major mysteries being whether neutrinos and antineutrinos are indeed the same particle. Discovering a rare, previously unobserved decay could potentially provide clarity on this question, and SNO+ is currently on the lookout for such a decay.
“It’s fascinating that pure water can be utilized to detect antineutrinos from reactors over great distances,” commented physicist Logan Lebanowski from the SNO+ collaboration and the University of California, Berkeley, in 2023 when the results were revealed.
“Extracting a handful of signals from 190 days of data was a significant effort for us, and the result is incredibly rewarding.”
The findings have been published in Physical Review Letters.
A previous version of this article was published in April 2023.
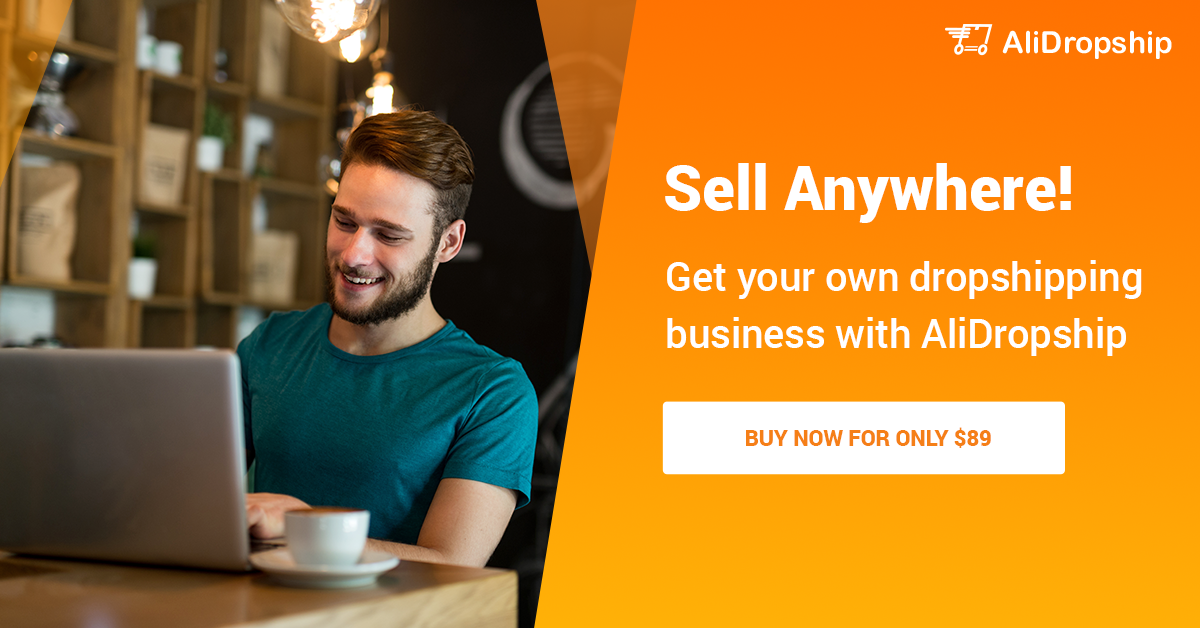